Solid tailings after supercritical CO2 extraction of lignocellulosic biomass as a source of quality biochar for energetic use and as soil improvement
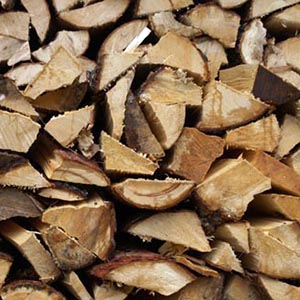
Accepted: 28 September 2022
HTML: 7
All claims expressed in this article are solely those of the authors and do not necessarily represent those of their affiliated organizations, or those of the publisher, the editors and the reviewers. Any product that may be evaluated in this article or claim that may be made by its manufacturer is not guaranteed or endorsed by the publisher.
Authors
Lignocellulosic biomass is a rich source of bioactive compounds extracted industrially from different parts of the plant. The extraction process generates residues containing 75 to 95% of the raw material, depending on the species. There is biochar among the many potential products of post-extraction residue’ processing. The research objective was: i) to evaluate the parameters of biochars derived from post-extraction bark, wood and bark and wood of four lignocellulosic species; and ii) to discuss the parameters in the context of biochar functionality as an energy carrier and soil improver. The residues were subjected to pyrolysis at the three temperatures, 170, 270, and 370°C, which correspond to the initiation of carbonisation, and two biochars that differ in the decomposition rates of hemicelluloses, cellulose, and lignin. On average, biochars had a high energy value owing to the increased total and fixed carbon and calorific value content by 77.0-78.4% DM, 64.6- 66.7% DM and 25.8-30.1 MJ kg–1, respectively. The higher quantity of ash after processing bark residues than wood residues implicates a lower energy value but, at the same time, the ash obtained is a better source of mineral compounds in soil fertilisation. Concerning using biochar as a soil improver, the biochars demonstrated lower hydrogen/carbon and oxygen/carbon molar ratios, indicating raised stability and resistance to the geochemical decomposition in soil. It was proven that the bark-based biochars had much higher concentrations of micro- and macronutrients, and a higher pH, while processed wood fractions resulted in higher concentrations of total carbon and fixed carbon in the biochar. The research results suggest that lignocellulose biomass extraction residues can serve as a valuable input material for biochar production.
How to Cite

This work is licensed under a Creative Commons Attribution-NonCommercial 4.0 International License.
PAGEPress has chosen to apply the Creative Commons Attribution NonCommercial 4.0 International License (CC BY-NC 4.0) to all manuscripts to be published.