Evaluation of microclimate in dairy farms using different model typologies in computational fluid dynamics analyses
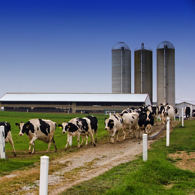
All claims expressed in this article are solely those of the authors and do not necessarily represent those of their affiliated organizations, or those of the publisher, the editors and the reviewers. Any product that may be evaluated in this article or claim that may be made by its manufacturer is not guaranteed or endorsed by the publisher.
Authors
Ventilation plays a key role in the livestock buildings since it is important to guarantee a comfortable environment and adequate indoor air quality for the animals. Naturally ventilated barns are usually characterized by high variability in the ventilation conditions. Moreover, the ventilation efficiency can be very different in different areas of a barn because of the different presence of the animals. On the other hand, appropriate ventilation is an essential requirement to ensure animal welfare and efficient and sustainable production since a proper ventilation is the most efficient way to remove undesirable air pollutants and to obtain a comfortable microclimate for the welfare of the animals. In this regard, the computational fluid dynamic (CFD) simulations represent a powerful and useful tool because they can be used to assess ventilation and microclimate conditions. In this context, the present study has the object to assess whether different CFD modelling approaches (i.e. model with animals modelled as obstacles with closed volume and model enriched with cows modelled as obstacles capable of exchanging heat with the surrounding air volume) show differences in relation to the climatic conditions inside a naturally ventilated dairy barn. The comparison of the results, set in terms of indoor air temperature and air velocity contours of the two different models, arises that if a precise definition of the microclimatic features is necessary, in order to correlate them with production parameters or assess animal welfare indexes, thermal simplification is not acceptable since can lead to completely misleading conclusions and incorrect evaluations. Then, only adopting CFD models considering the animal thermal behaviour is possible to obtain effective information both for the proper barn system management and for the creation of useful tools driving the farmers' choices.
How to Cite

This work is licensed under a Creative Commons Attribution-NonCommercial 4.0 International License.