3D-printed biological habitats for the protection and persistence of Rhizobia species in compacted soils
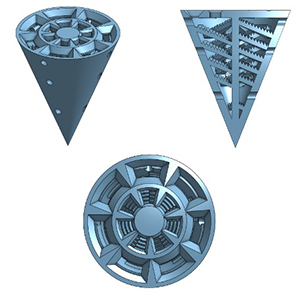
All claims expressed in this article are solely those of the authors and do not necessarily represent those of their affiliated organizations, or those of the publisher, the editors and the reviewers. Any product that may be evaluated in this article or claim that may be made by its manufacturer is not guaranteed or endorsed by the publisher.
Authors
Microorganisms in soils are responsible for many ecosystem services. However, in degraded soils, microbial abundance and function are limited, compromising several biologically facilitated processes. Inoculating soils with desirable microbes can help to re-instate or initiate a viable functioning microbial community. However, establishment success is reliant on the survival of the microorganism in an adverse environment. In this proof-of-concept study, artificial microbial refugia have been developed using resin and light-emitting diode array (LED) 3D printing technology. We assessed whether the artificial refugia, termed a Rhiome, would support better microbial growth in degraded soils. Soil compaction, a form of soil degradation, and Rhizobium, an important microorganism for global agriculture, were selected as the use case application for this assessment. Different materials, together with resin, were assessed for their suitability as a 3D printing material and for supporting rhizobial growth. The best result was found in materials constructed with a combination of polylactic acid (PLA) resin, yeast extract, and mannitol. In a soil compaction experiment with inoculation of rhizobia, the addition of Rhiome significantly increased bacterium survival in the compacted soil to a level similar to, or higher than, the rhizobial loading in non-compacted soils. In addition, augmentation of the resin with yeast extract and mannitol increased Rhizobium growth significantly compared with the Rhiome constructed only with resin. These results indicate that the Rhiome was highly beneficial for instigating and maintaining significant rhizobia survival and growth in compacted soils. Further work, including near-to-field assessments, is required to assess Rhiome performance in various applications and to refine material properties relative to important context-specific performance metrics such as degradation rate. We propose the Rhiome concept as a promising asset in the toolbox for soil ecological restoration as a means of improving soil resiliency.
How to Cite

This work is licensed under a Creative Commons Attribution-NonCommercial 4.0 International License.
PAGEPress has chosen to apply the Creative Commons Attribution NonCommercial 4.0 International License (CC BY-NC 4.0) to all manuscripts to be published.